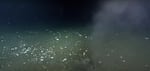
The Pythias Oasis, shown here in a video screenshot, is a plume of mineral-laden seawater seeping from the seafloor off the coast of Newport, Oregon, at an unprecedented rate. While it doesn’t mean that an earthquake is imminent, the seep could give researchers more insight into how the Cascadia Subduction Zone functions.
Deborah Kelley/University of Washington/U.S. National Science Foundation/Canadian Scientific Submersible Facility
About 50 miles off the coast of Newport, hot, mineral-laden seawater is seeping out of the ocean floor at an unprecedented rate. Researchers at the University of Washington say the liquid acts as a kind of lubricant between tectonic plates, possibly contributing to pressure buildup along the plate boundary. While it doesn’t mean that an earthquake is imminent, the seep could give researchers more insight into how the Cascadia Subduction Zone functions. Joining us with more details is Evan Solomon, an associate professor of oceanography at UW.
The following transcript was created by a computer and edited by a volunteer:
Dave Miller: This is Think Out Loud on OPB. I’m Dave Miller. Hot, mineral-laden seawater is gushing out of the ocean floor about 50 miles off the coast of Newport. It’s at the Cascadia Subduction Zone, in a site known as a seep. But researchers at the University of Washington say the flow of this liquid is way higher than from other seeps. The Cascadia Subduction Zone, for folks who have not gotten the message yet, is the meeting of two tectonic plates that has led to massive earthquakes every 500 years or so. The last one was in the year 1700.
This huge seep doesn’t mean that an earthquake is imminent, but the new discovery could give researchers more insight into how the Cascadia Subduction Zone functions. Evan Solomon joins us with more details. He is an associate professor of oceanography at the University of Washington. Welcome to Think Out Loud.
Evan Solomon: Thank you. Thanks for having me.
Miller: So, when was this particular site first discovered?
Solomon: The site was discovered in 2014 on kind of a transit of opportunity. There’s work that’s been conducted at a site called Hydrate Ridge, which is also offshore [of] Newport. And it’s one of the most well studied sea sites in the global oceans. It’s part of the 00I Cabled Observatory. And there’s a fiber optic cable that runs off of Newport and there’s instrumentation, collecting data in real time all the time at Hydrate Ridge. And so every year researchers go out there to do operations and maintenance on the observatory and when they run into bad weather and can’t use the remotely operated vehicle to dive on that site, the ship is expensive so they do mapping with the ship when the weather’s bad.
This site was actually discovered during a period of bad weather where they couldn’t do work at Hydrate Ridge and an undergraduate at UW, his name is Brendan Phillip, he’s no longer an undergraduate but he was at the time [and] led the survey of this, of the Sonar survey south of Hydrate Ridge. And that’s when they discovered this new sea site, was during a routine kind of hydrographic survey, a routine sonar survey.
Miller: And then you and others went back to learn more about it. How do you actually figure out what’s happening at a site like this at the bottom of the ocean floor?
Solomon: The seep is one of many along the Cascadia Subduction Zone. We identify them usually in ship based sonar, so during multi beam mapping, and we could pick up the gas emissions really well. People have looked at the archive data and the large database of sonar surveys along Cascadia and there’s a minimum of 340 seep sites, they’re all over the place. But this was one that wasn’t in the database. So there was a follow-on dive with a remotely operated vehicle in 2015 that Brendan Phillip was [on], who is now at that point a graduate student here [and he] led that dive.
What they basically did is they knew where the gas emissions were coming from at the sea floor and they brought the ROV down to investigate that. And as they got closer and closer to the site where they thought the seep emissions were coming from, they started getting water column anomalies. They started seeing an increase in temperature in bottom water and they started seeing a decrease in salinity, which is quite unique. Usually the flux of water from these sites isn’t very high. And then as they got closer and closer, they started getting a stronger signal and then what they saw was water gushing out of the sea floor at a really extreme rate, it was like a fire hose of water coming out of the sea floor, completely spectacular. So I wasn’t on that expedition, but once they returned and told me about the site, I got very excited. And it was at that stage we put in a full scale proposal to the National Science Foundation to do more in-depth focus study on this new seep site.
Miller: Can you give us a sense for how much faster water was gushing out of this seep site than in the other 300-plus that had been identified?
Solomon: Sure. So seep sites are regions of . . .they’re basically sea floor manifestations of gas and water emissions from deeper within the sediment column. That’s where the fluids are emitted at the sea floor. And seep sites don’t have to be associated with fault zones. There’s seep sites, and non-active margins - an active margin being like a seduction zone - like the US East Coast. But many of the seep sites typically coincide with fault zones, so zones where there’s faults within the subsurface that aids in fluid emissions. And in general, in a seduction zone environment like Cascadia, the rate of water flow along these fault zones that we measure at seats are on the order of two to 10 centimeters per year. So geologically, that’s actually quite fast. But if you’re sitting at home thinking about something moving only 10 centimeters per year, that’s not very fast.
Miller: The water is moving 10 centimeters a year, or the rocks are?
Solomon: No, the water is.
Miller: Meaning it’s like it’s not moving.
Solomon: Again, over long time scales actually, that’s quite fast. But yeah, it doesn’t seem like much. That’s what a general kind of flow rate is, a fluid flow rate at a seep site. At this particular seep site the flow rate is 90 million times faster than what we generally observe seeps globally, so it’s incredibly fast. The rate of water emission at the seafloor is about a half a liter per second, which is roughly eight gallons per minute. You would fill up a bathtub in about four minutes with the rate of discharge or fluid flow at this particular seep site, so it’s super fast. Something that [is] the highest rates to my knowledge that have been observed worldwide.
Miller: How do you explain that? It’s the way you’re describing it, it doesn’t even seem like “seep site” is the accurate term, it’s more like a “gusher site.” But what makes this one different?
Solomon: That’s funny you say that. So the original name for the site was “The Gusher”, that Brendan named it. Then he renamed it the Pythias Oasis seep site. But what is driving this is that, what drives the transport of water in the subs seafloor environment are pressure gradients. So you have a region of elevated pressure flowing in and then another region where we have lower pressure that permits the flow of fluid from one place to the other. The other thing that’s really important is the permeability of that sediment. The permeability is basically the interconnectedness of pore space. So if you’re in a hose where there’s an open hole, then you can flow really fast. But if you start packing that hose with sediment or something like that, then that’s gonna decrease the permeability and decrease the flow rate, even though the pressure is the same, right? And so in this particular site that we looked at, the permeability has to be very high to permit these flow rates that we’re observing.
Miller: So can you give us a sense for the marine life around there? Big or small, around this vent? I’m asking this because not too long ago, we talked about the huge perfusion of bacterial life around sea vents, and folks can find that conversation at our website, OPB.org/ThinkOutLoud. But what do you see or what does the remote-operated vehicle see around there?
Solomon: These seep sites are spectacular. They’re oases kind of in the desert, at least for the seafloor environment. If you’re away from a seep site, let’s say, on the continental margin, it just looks like mud. There’s a very robust microbial community within the sediments, but it’s not something that we see, the density isn’t high enough. When you get to a seep site, because the composition of the fluids is different than seawater, and you have all these organisms that are chemosynthetic and so they survive from chemical reaction rather than sunlight. Then you have these oases of communities at the seafloor. And so that’s kind of ubiquitous at these seep sites globally, but at this particular site there are fields of clams, there are thick bacterial mats, probably similar to what people that you were talking to observed at hydrothermal systems. Those kind of make the base of the food chain, then you start getting macro fauna like crabs and sea stars and other organisms that are then feeding on those organisms. It looks like mud as you’re away from the seep site but once you get to the seep site, it’s just teeming with life.
Miller: Let’s turn to the question that I think has been top of mind for a lot of people, especially given some, I guess overly sensationalized headlines about you at work: this is the connection between this gushing stream of water and the “big one” that so many of us are rightfully scared of. So, first of all, what’s the connection between this superheated water venting out at the bottom of the ocean and the movement of the plates themselves?
Solomon: Sure, I wanna say two things about that. The first is, one really excellent or great thing about this site is, because the water is coming at a high rate, we get a really pristine sample of that fluid, so we can sample that fluid and then look at the chemical composition of that fluid. And from the chemical composition, we can then say what temperature and depth the water is migrating from. And so based on the composition of the fluid, we’re estimating that the fluid originates from 180 to 220 degrees Celsius temperature, which in Fahrenheit’s around 350 to 430 degrees Fahrenheit. So that’s coming from really great depths and using that temperature we can say that the fluids have migrated from four kilometers below the seafloor, which is roughly 2.5 miles.
Miller: And it can be that hot, way above boiling because it’s under such pressure?
Solomon: Correct, it’s under pressure. And then as it’s migrating to the surface, it’s losing a lot of that heat. There’s also a lot of mixing with ambient fluids that are shallower in the system that cools it down. So by the time it gets to the sea floor, it’s not at 350 degrees Fahrenheit, it’s at a much lower temperature. But it retains those chemical characteristics which allows us to invert that to understand what temperature the fluids originated at, which is quite useful and exciting.
A depth of 2.5 miles puts us at the plate boundary itself. The Juan de Fuca plate and the North American plate are on the plate boundary in this region. That depth is well beyond the depth that we can ever sample, at least with current technology, even [with] scientific ocean drilling we can’t drill to a depth of 2.5 miles within one of these subduction systems.
What these fluids are doing is providing is kind of an in situ sample outside of where we can actually sample, in situ providing information on the chemical composition of those fluids and fluid rock reactions that are occurring within the plate boundary itself, which is quite unique and it’s because this fluoride is so high that we’re getting a relatively pristine signal. That didn’t answer your question.
Miller: No, but it shows something super exciting, all of which was just based on a weather accident. I mean, you wouldn’t even have known this if the weather had been good that day.
Solomon: Correct. That is totally correct because gas emission sites do not typically have this kind of water flow rates, and so it was really fortuitous.
Miller: Ok. But so the short version of what you just said is that you now have access to learn more about the chemical composition of this water that is at the meeting point of these two plates, four kilometers under the seabed. What can you learn from that?
Solomon: So, there’s a couple of things; I don’t want to spoil our next study. We’ve been investigating those foods in detail now and we’re learning quite a bit about what is in the fault zone at those depths and other information. That’s stuff that’s still in progress in terms of analysis here in my laboratory. But to kind of address the question that you originally had is, how is this important or help our understanding for processes that occur in the Subduction Zone, [it] is the location of the seep site itself. This particular seep… like I said, many seeps are faults associated and there are a lot of faults within subduction zones that intersect the plate boundary at depth. And many of those faults are called thrust faults, which have very long distances.
This particular seep site sits on top of one of the strike-slip faults in the Cascadia Subduction Zone. A good example of the strike-slip fault would be the San Andreas Fault in California. These certainly are not as long as the San Andreas Fault, but you have motion side by side, but not up or down along a strike-slip fault, and they tend to be vertical. They’re a much shorter transport distance to get from where it’s intersecting the plate boundary to the seafloor in one of these strike-slip folds. And so Pythias is sitting there and it’s indicating that these strikes-faults, this one happens to be called the Alvin Canyon Fault, is highly, highly permeable and is allowing really efficient drainage of fluids from depth along the plate boundary in this region. And that is something that we, [or] I at least hadn’t considered before the discovery of the site. Usually I’m focused on thrust faults because they’re more ubiquitous. And this is the first time that I’ve actually really had the opportunity to investigate the hydrogeology of one of these strikes-faults.
Miller: In a press release about the most recent finding you noted that this liquid can act as a lubricant; and correct me when I get this wrong, but it could act as a lubricant that enables a more frictionless slippage between the plates, so am I right so far?
Solomon: Yeah.
Miller: Ok. So if that’s the case and if this liquid is gushing out, how does it not mean that the plates are more likely to lock up and then catastrophically move as the liquid dissipates?
Solomon: Again, this finding doesn’t change our assessment of risk or earthquakes within the Cascadia Subduction Zone. It’s not because of this site that we’re gonna have any kind of earthquake or something like that. But the things that matter for locking, in the Cascadia Subduction Zone we have locking between the two plates from Canada, from British Columbia all the way to Northern California. And what drives that locking is the frictional characteristics of the material; so what kind of rocks or sediment are within the fault zone, but also the pore fluid pressure. And that’s the pressure between the sediment grains within the fault zone, or just below the fault zone, is actually another thing that’s very important for the locking process.
In general, if you have really high pore fluid pressure that decreases the stress in the fault, you can have more stable or aseismic sliding or things called slow slip events. And those are slip along the plate that doesn’t generate an earthquake, either continuous at the rate that the plate is abducting or kind of semi-periodic, very slow rates of slippage along the plate boundary. If the pore pressure drops, then the stress along the fault zone increases and that contributes to the locking. So it’s not just the pore fluid pressure, it’s also the frictional characteristics of the material. But they’re both intimately related to the locking process.
So as you said, this drainage of fluid that is occurring at Pythias and along the strikes of fault, is decreasing the poor fluid pressure along the plate boundary and contributing to the locking that we observe. And actually in this particular location, we see, right where these faults tend to be, we see an increase in consolidation and changes in locking in this particular region. And so what it’s telling us is that these fault zones are intimately related to drainage along the plate boundary or allowing fluids to escape, which is intimately related to the kinds of patterns of stress that we see along the fault zone.
Miller: Evan Solomon, thanks very much for joining us. That is Evan Solomon, an associate professor of oceanography at the University of Washington.
Contact “Think Out Loud®”
If you’d like to comment on any of the topics in this show, or suggest a topic of your own, please get in touch with us on Facebook, send an email to thinkoutloud@opb.org, or leave a voicemail for us at 503-293-1983. The call-in phone number during the noon hour is 888-665-5865.